In any sensing solution, it is important that the sensors used can produce high quality and accurate signals. To achieve this quality, care needs to be taken in choosing the best possible components. The Shimmer Engineering team puts great effort into ensuring that our devices can produce the high quality needed by our customers. Through our hardware design process and the calibration procedures that we provide, the Shimmer3 produces signals which are ideal for highly accurate and reliable sensing solutions.
Noise
Noise is the random deviation of a sensor signal. It is an unwanted characteristic of all electronics that affects the quality of the output signal. Noise can arise from both internal and external sources. Internal noise is caused by passive elements within electronics, such as thermal noise due to the random motion of electrons in resistors. External noise, or atmospheric noise, is naturally occurring interference from the Earth’s atmosphere.
Minimising noise is an important aspect in the design of electronics. The low-noise accelerometer on the Shimmer3 is a KXRB5-2042 analog accelerometer from Kionix, with a noise density of 45 µg/sqrt(Hz). This compares favourably to other devices, like Google Glass, for example, which relies on an MPU-9150 accelerometer from InvenSense with a noise density of 400 µg/sqrt(Hz) – an order of magnitude greater than that of the Shimmer3 low noise accelerometer.
To illustrate this effect graphically, if sensors with these levels were used to capture an image, the Shimmer3 equivalent sensor would produce the image on the left hand side of Figure 1, whilst the Google Glass equivalent would produce the image shown on the right hand side of Figure 1. The Shimmer3 equivalent can be seen to produce a high quality, clear, sharp image.
Quantisation
To convert an analog signal to a digital one, the entire range of the analog signal is divided into a finite number of quantisation levels, with each quantisation level representing a discrete value. The resolution of an analog-to-digital converter indicates the number of these quantisation levels. The error associated with this conversion is called the quantisation error. A higher resolution means a greater number of quantisation levels and leads to a lower quantisation error and a more accurate representation of the original analog signal.
The Shimmer3 gyroscope sensor, which is on the MPU-9150 chip from InvenSense, has 16-bit resolution. For comparison, the gyroscope sensor on the Samsung S3 is provided on the LSM330DLC chip from STMicroelectronics and has a maximum resolution of 12 bits.
Resolution is graphically represented in Figure 2. A 16-bit resolution image is shown in on the left hand side of the figure, along with a 12-bit resolution representation of the same image for comparison. The inset in each case provides a zoomed in image of the letter ‘m’, to highlight the effect of the difference in quantisation. The high resolution used by the Shimmer3 gyroscope sensor produces a smooth, clear image, whilst, in the 12-bit resolution image, the quantisation levels are evident.
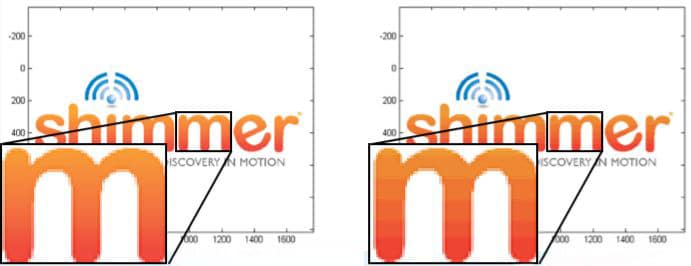
Calibration
Bias, alignment and sensitivity errors are effects that occur to varying degrees in all sensors, especially inertial sensors, and they effect the accuracy of the signals produced by those sensors. To overcome these variations, calibration techniques can be used.
Bias
Bias is a non-zero offset measured by a sensor when the input is zero. For a gyroscope, a bias error will mean that, when the device is stationary, a non-zero angular rotation will be output from the device. Without correction, this value would cause signal processing algorithms interpreting the data to treat the device as though it were in motion, when this is not the case, or to produce rotational velocity readings that are too high or too low when the device is in motion.
For illustrative purposes, Figure 3 shows an example of how an image is affected by bias errors. The RGB (Red, Green, Blue) sensors in the image on the right hand side of the figure have been subjected to positive bias offsets, meaning that the sensed image is not a faithful representation of the true image, depicted on the left hand side of the figure, but, instead, has incorrect levels of saturation due to the increased amplitude of each RGB component.
Alignment
Alignment deviations can also occur in sensors. The inertial sensors on the Shimmer3 are tri-axial sensors, which measure the phenomenon of interest in three dimensions. Alignment error can mean that a pair of axes are fully swapped (e.g. the sensor’s X-axis is aligned with the ‘true’ Y-axis and vice versa) or that there is a partial misalignment, such that the measurement from a given axis is a vector which is not parallel with the assumed axis direction. For a tri-axial sensor, the effect of misalignment is a rotation of the sensing axes in three-dimensional space.
To illustrate this effect visually, Figure 4 shows the effect of alignment error in image processing. In the figure on the right hand side, the RGB axes have been misaligned, such that the R-axis of the sensor is aligned with the B-axis of the original image (shown on the left hand side of the figure), the G-axis of the sensors is aligned with the R-axis of the original image and the B-axis of the sensor is aligned with the G-axis of the original image. The effect is a transformation of the colour space.
Sensitivity
The sensitivity of a sensor relates to how much the output of the sensor changes when the measured quantity at the input changes. A sensor can be over- or under-sensitive, meaning that the resultant signal will be amplified or attenuated, respectively, relative to the ideal output. For inertial or other sensors, sensitivity errors in one or more sensed components will mean that the changes in the output signal will not provide a true representation of the changes in the sensed phenomenon.
Once again, a graphical example is provided to illustrate the effect of sensitivity. In the image on the right hand side of Figure 5, the sensitivity of the red (R) component has been reduced, relative to the ideal value. The effect is that the green (G) and blue (B) components appear stronger, relative to the red components, distorting the colour of the colour vectors.
To counteract the above effects of bias, alignment and sensitivity errors, Shimmer provides the Shimmer 9DOF calibration application, which improves the quality of the signals produced by the inertial sensors.
Comparison: Shimmer3 vs. Samsung S3
Table 1 and Table 2 below compare the performance of the Shimmer3 gyroscope (MPU-9150) with the Samsung S3 gyroscope (LSM330DLC) and the Shimmer3 low-noise accelerometer (KXRB5-2042) with the Samsung S3 accelerometer (LSM330DLC). These results were experimentally measured by the Shimmer team, with data sets for both the Shimmer3 and the Samsung S3 collected simultaneously to ensure a like-with-like comparison. The devices were placed side-by-side on a flat surface, with the Z-axis of both accelerometers pointing in the direction of the gravity vector, and they remained stationary for the duration of the data collection.
The Shimmer3 gyroscope demonstrates better noise performance than the Samsung S3 gyroscope, as seen by the lower standard deviation value for the Shimmer3. For the accelerometer signals, the noise performance for both devices is similar in this experiment.
The average error of the Shimmer3 gyroscope and accelerometer signals is seen to be less than that for the Samsung S3. This is a result of the calibration of the Shimmer3 device, using Shimmer 9DOF Calibration application, which successfully eliminates the bias, sensitivity errors and alignment errors of the device.
For more information about sensor calibration or for information about available Shimmer products and services, feel free to contact us on info@shimmersensing.com